Dr. Vandana Shiva was the keynote speaker at two events on March 15 and 16, in Mexico City, in support of the Mexican Government’s stand against glyphosate and GMO Maize.
Regeneration International was one of the organizers of these events in partnership with Navdanya International, the Organic Consumers Association, Vía Orgánica, and Sin Maíz No País.
On March 15, the Department of Environment and Natural Resources hosted a scientific forum on the “Protection and conservation of biodiversity in the regions considered as centers of origin of species.” Dr. Shiva was the keynote speaker, and Dr. André Leu was the following speaker on the importance of conserving both agricultural and endemic biodiversity.
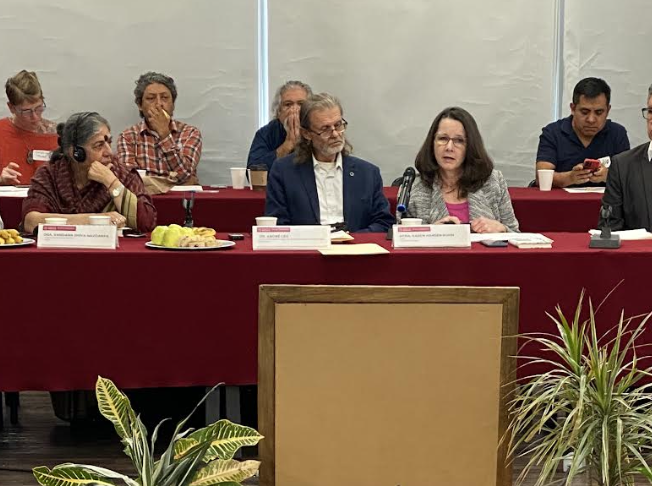
Caption: Dr. Vandana Shiva (Navdanya), Dr. André Leu (RI), and Karen Hansen (IATP) speaking at The Department of Environment and Natural Resources hosted a scientific forum
The following day, March 16, was a well-attended public event with many speakers. Dr. Shiva gave the closing keynote address to a very receptive and appreciative crowd. Dr. Mercedes Lopez, from Vía Orgánica, gave a rousing speech on the importance of maize to Mexican culture and the need to not contaminate the traditional varieties with GMOs, as this would destroy the fact that Mexico is the center of origin and diversity for this critical food staple.
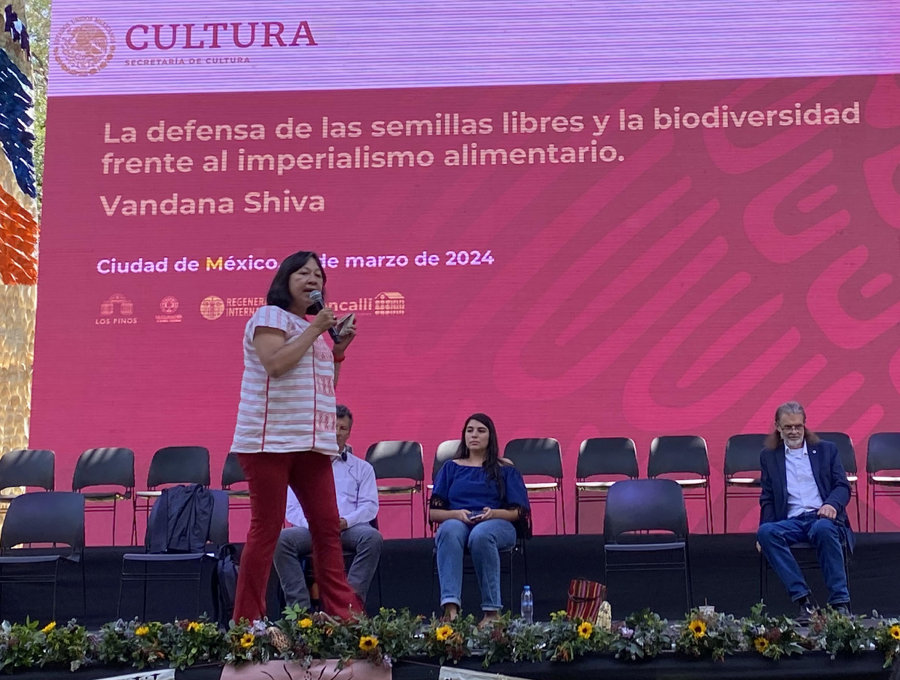
Caption: Dr. Mercedes Lopez speaking at the well-attended public event on March 16
I spoke on the risks and damages associated with transgenic corn and glyphosate, presenting scientific evidence of the harm it is causing to human health.
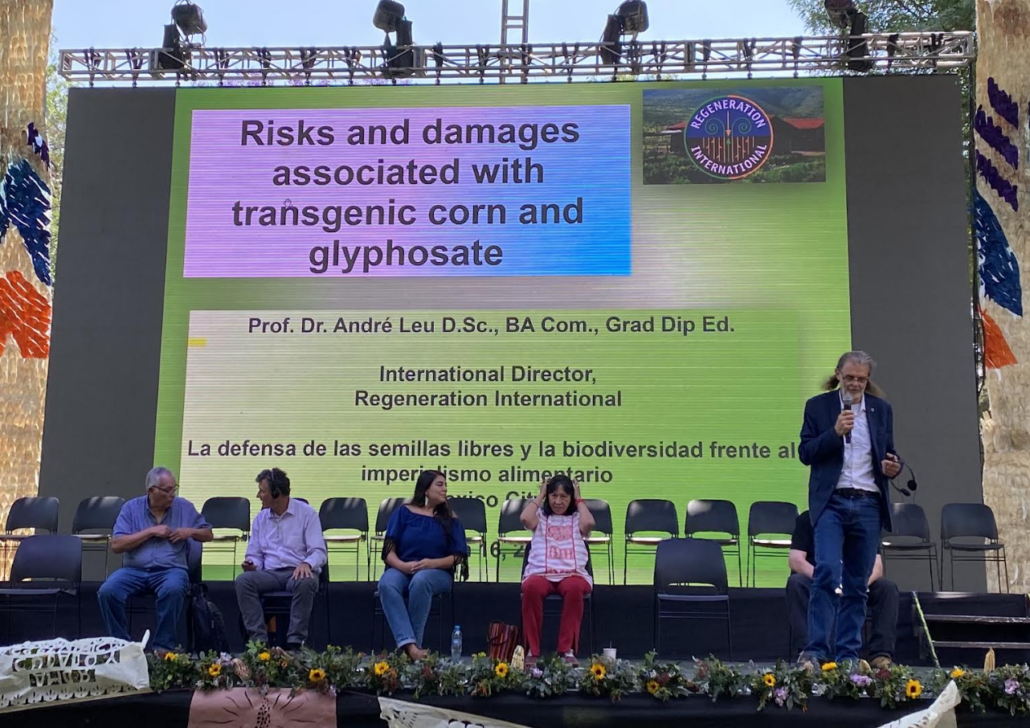
Caption: Dr. Andre Leu from Regeneration International Speaking at the public event
I was one of the many scientists who assisted Mexico in developing a science-based case against US bullying, which aimed to force them to reverse their decision to ban glyphosate and GMO maize.
US Government Poison Cartels Puppets Bully Mexico over its Sovereign Right to Ban Glyphosate and GMO Corn
Mexico announced plans to ban glyphosate and GMO Maize in 2023. Bayer-Monsanto and Dow launched 43 lawsuits in Mexico attempting to overturn the presidential decree.
The GMO/pesticide cartels, fearing that Mexico will set a precedent for other countries to enact similar restrictions, are puppeteering agencies and officials within the U.S. government to pressure Mexico to abandon its plans. This is not the first time the German-based Bayer-Monsanto has used its captured U.S. government officials and agencies to act on its behalf. In 2019, the corporation succeeded in using U.S. officials to pressure Thailand to reverse its ban on glyphosate.
According to Reuters, the new U.S. agriculture trade chief, Doug McKalip, gave Mexico until February 14, 2024, to respond to the U.S. demand to justify the science behind the GMO maize and glyphosate ban.
Mexico responded in March by releasing a formal rebuttal of U.S. efforts to overturn their ban.
The government produced an 189-page scientific report stating, “Mexico has legitimate concerns about the safety and innocuousness of genetically modified corn … and its indissoluble relationship with its technological package that includes glyphosate,” the government’s report states. It showed evidence that the use of pesticides causes serious health effects.
Mexico states that there is “clear scientific evidence of the harmful effects of direct consumption of GM corn grain in corn flour, dough, tortillas and related products.”
The Scientific Evidence That Justifies Mexico Banning GMOs and Glyphosate
There are an enormous number of published scientific studies showing that GMOs and their associated pesticides are responsible for multiple serious health problems for people, animals, and the wider environment.
The widespread adoption of GMO crops in the U.S. has resulted in a massive increase in the application of glyphosate, the active ingredient in Roundup, as the primary method of weed control.
The Only Credible Peer-reviewed Lifetime of GMOs and Roundup
Only one credible, independent, non-industry funded, peer-reviewed lifetime feeding study of GMOs and Roundup exists. It found that mammary and other tumors, liver and kidney damage resulting from regular exposure to minute amounts of Roundup and/or a diet containing GMO corn – similar to the typical exposures people get from food.
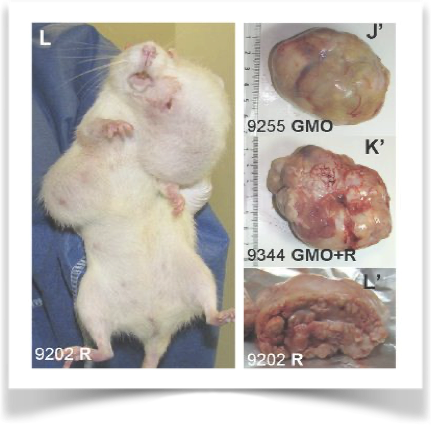
The image above shows a rat with large mammary tumors caused by consuming glyphosate at the usual levels found in food. The tumors on the right-hand side, starting from the top, result from eating GMO corn, GMO corn with Roundup, or just Roundup. (Seralini et al.)
All the female rats in the study that were fed GMOs and/or Roundup (Treated Group) developed mammary tumors and died earlier than the rats who were fed non-GMO food without Roundup (Control Group), except for one rat who died early of an ovarian tumor.
Treated males presented four times the number of tumors that were large enough to be felt by hand than the controls, and these occurred up to six hundred days earlier.
The International Agency for Research on Cancer (IARC)
The International Agency for Research on Cancer (IARC) has given glyphosate the second-highest rating for Cancer – Group 2A.
This means it causes cancer in animals and has some evidence of cancer in humans, most notably non-Hodgkin’s lymphoma.
A study by Flower et al. examined the levels of cancer in the children of people who sprayed glyphosate for weed control. They found that these children had increased levels of all childhood cancers, including all lymphomas, such as non-Hodgkin’s lymphoma.
A case-controlled study by Swedish scientists Lennart Hardell and Mikael Eriksson also linked non-Hodgkin’s lymphoma to exposure to various pesticides and herbicides, including glyphosate. The link between glyphosate and non-Hodgkin’s lymphoma has resulted in significant court cases, most of which Bayer-Monsanto has lost and awarded millions of dollars to the victims.
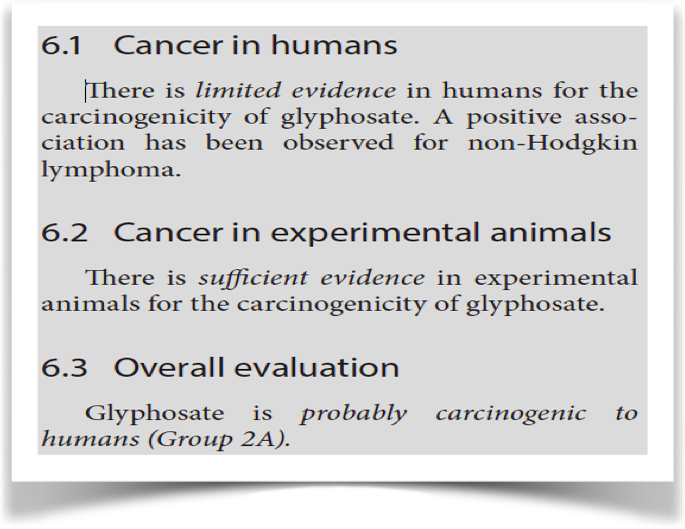
Genetically engineered crops, glyphosate, and the deterioration of health in the United States of America
Dr. Nancy Swanson, myself, and co-authors Jon Abrahamson and Bradley Wallet published a peer-reviewed paper, “Genetically engineered crops, glyphosate and the deterioration of health in the United States of America,” showing how glyphosate and GMOs are linked to over 20 diseases in the U.S. The study searched US government databases for genetically engineered crop data, glyphosate application data, and disease epidemiological data. This was correlated with numerous diseases linked to the increased use of glyphosate and GMOs. A standard accepted statical analysis showed that the odds of glyphosate and GMOs not being the cause of these diseases was 10,000 to 1. On top of these, numerous studies are confirming the link between GMOs and glyphosate with these diseases.
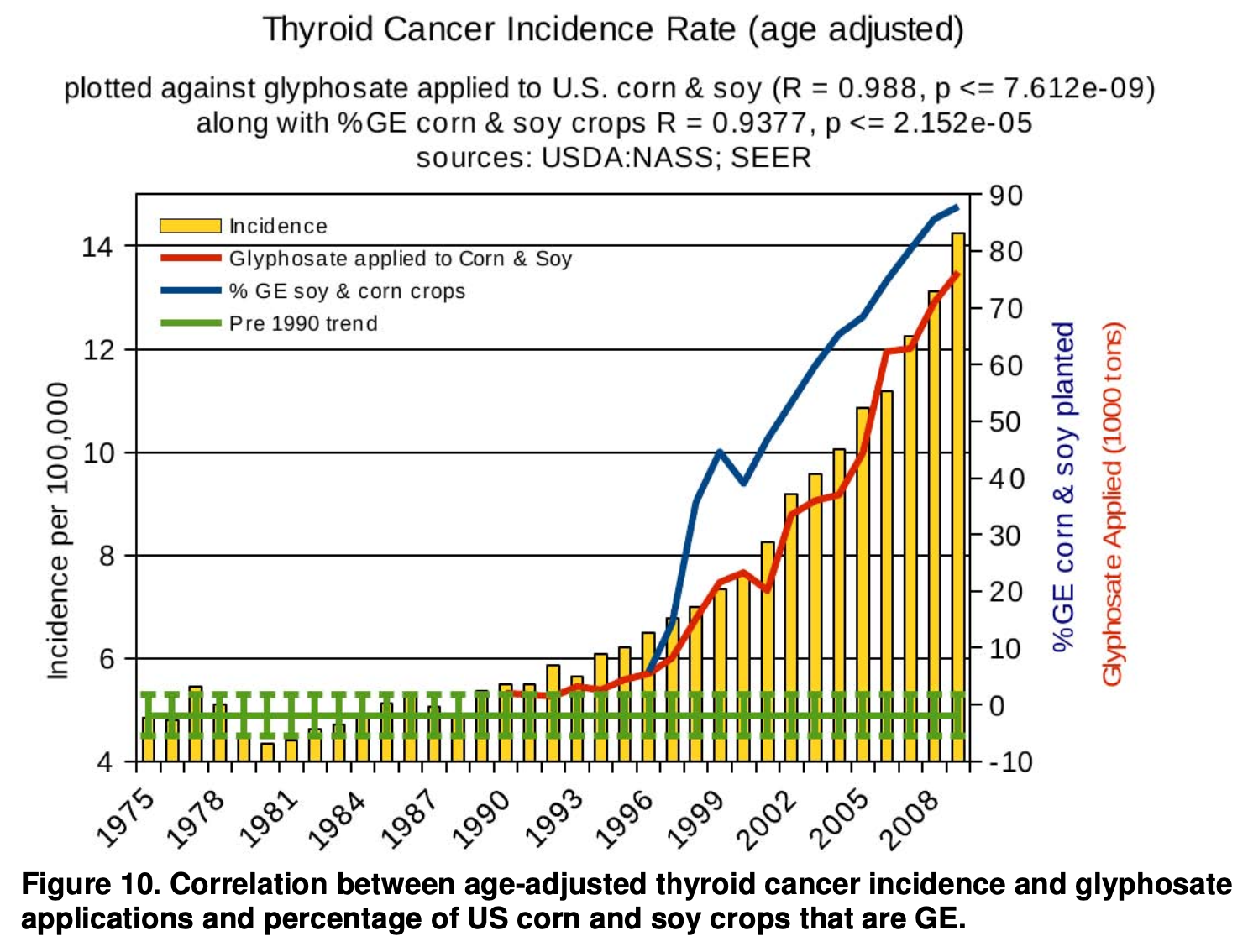
We compiled this data into graphs showing the increase in diseases, glyphosate, and GMOs. We also added trend lines in green to show that these diseases are increasing due to the increased use of genetically engineered (GE) corn and soy and glyphosate.
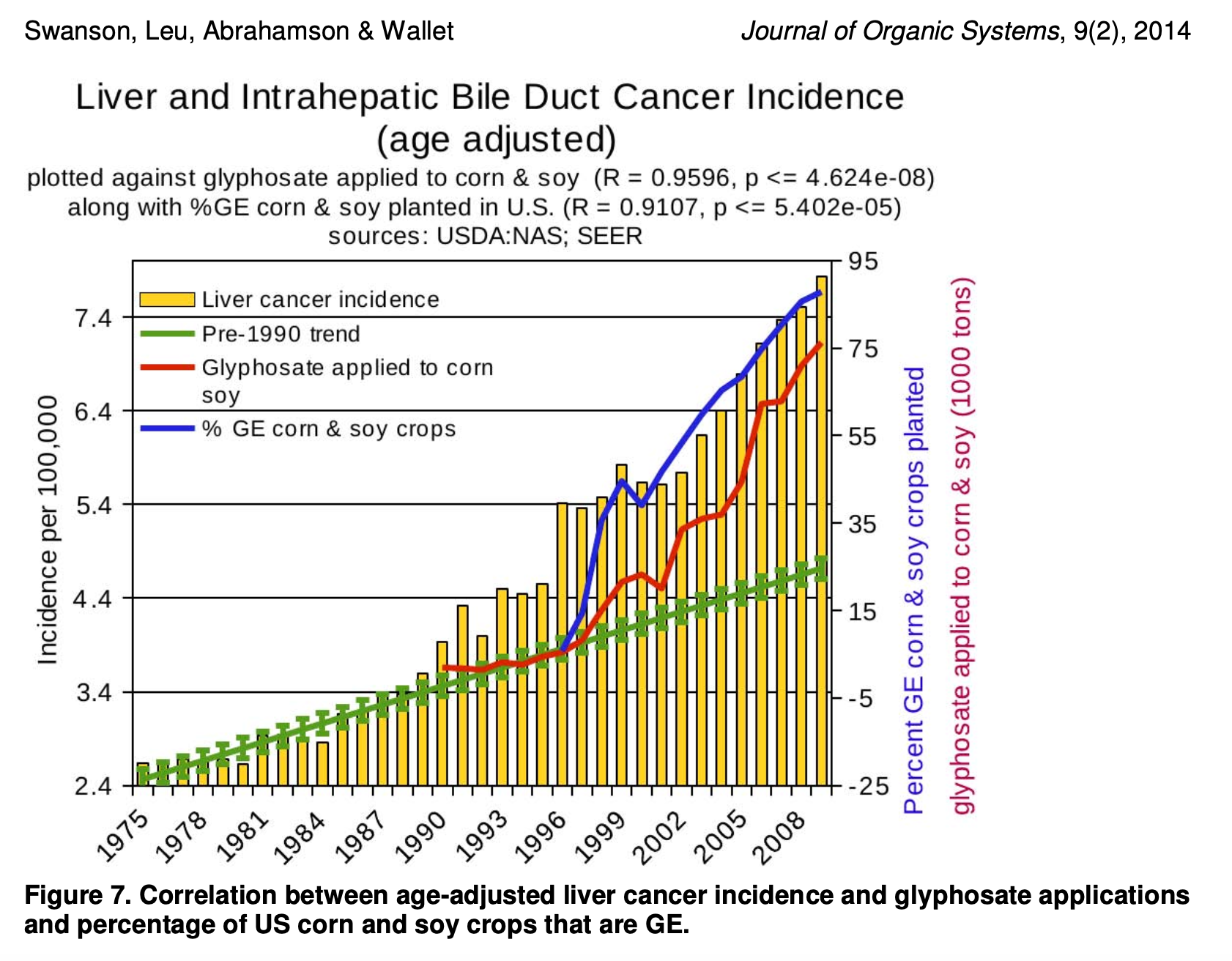
Autism and Dementia
Autism and dementia have reached epidemic proportions in the U.S. The gap below clearly shows the link between the massive increase in the use of glyphosate and GMOs since the 1990s and the increase in these diseases.
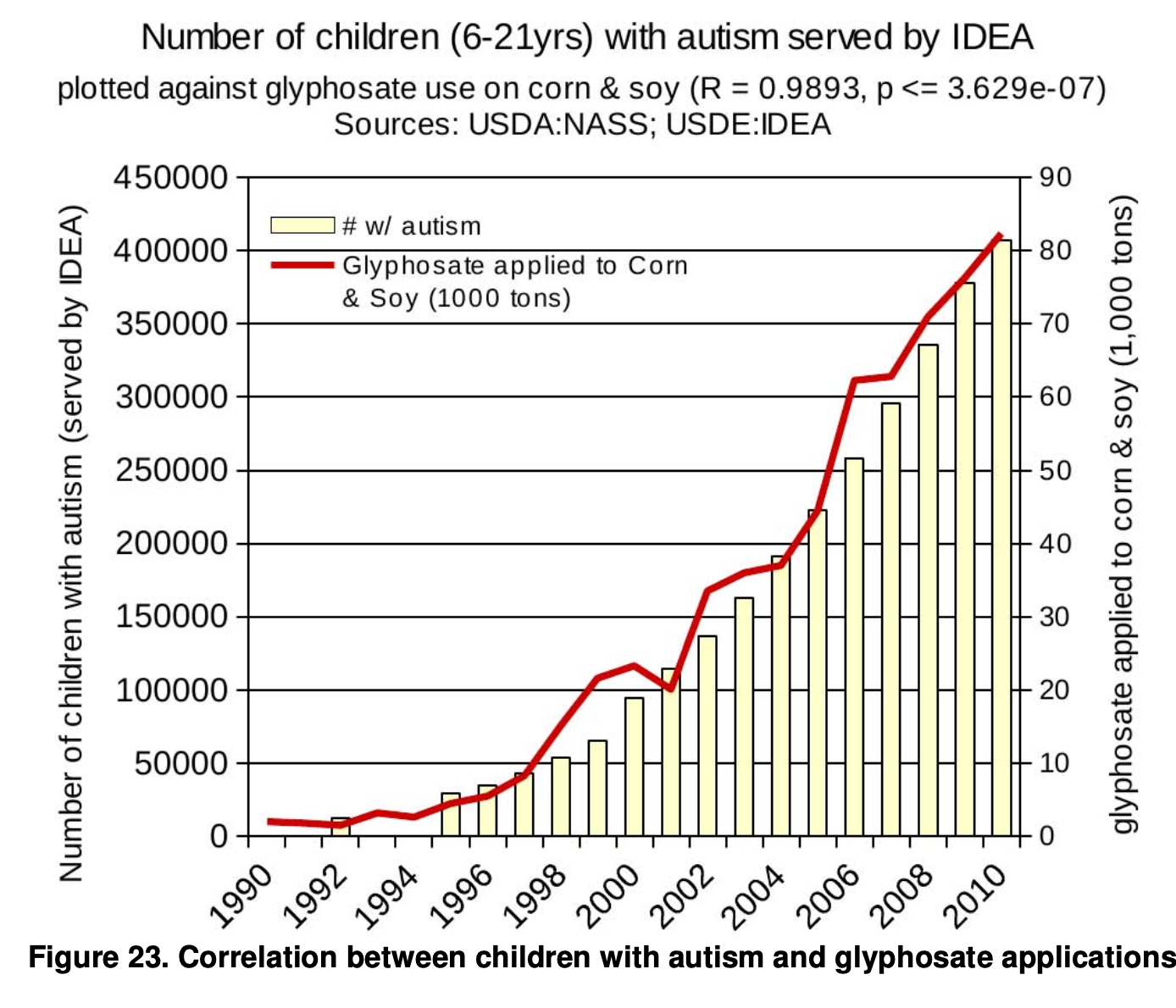
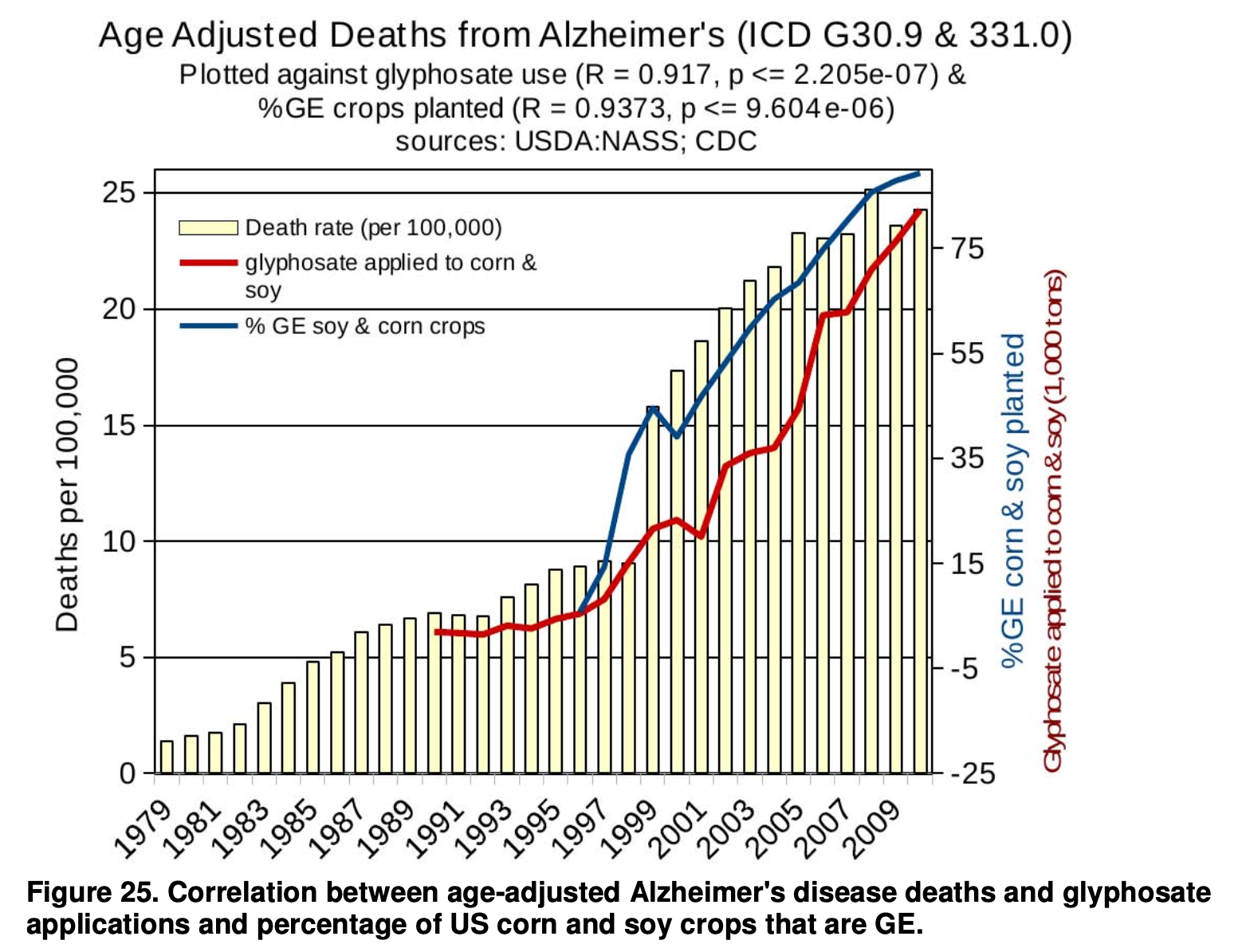
Researchers have shown how exposure to minute amounts of glyphosate damages the normal development of nerves.
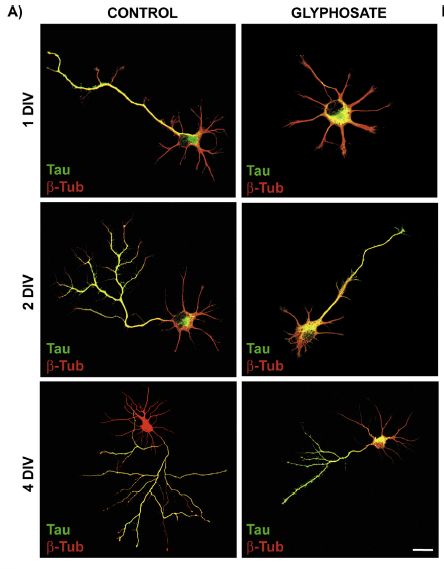
The image above shows how glyphosate damages nerve development. The glyphosate-exposed cells had shorter and unbranched axons, (the long extended ‘arms’ of the nerve) and less complex dendritic arbors (the smaller ‘fingers’ coming out of the body of the cell). It is clear from the image that the cells exposed to glyphosate do not develop properly and, therefore, cannot work effectively.
The scientists identified the cause by which glyphosate affects nerve development and stated that it cannot be reversed. The major concern is that the brain is the largest collection of nerves in the human body and is still developing in unborn, newborn, and growing children. Exposure to small amounts of glyphosate in food can adversely affect the brain’s normal development, leading to the suite of major issues that we see in children, such as autism spectrum, bipolar spectrum, ADHD, and other developmental and behavioral issues.
Adult brains are constantly renewing brain cells. These nerve cells are also adversely affected by glyphosate. The graph above shows a very strong link between the increase in glyphosate and deaths from dementia.
Endocrine Disruption – Disruption to Hormones
Gasnier et al. reported endocrine-disrupting actions of glyphosate at 0.5 ppm. According to the authors, this is “800 times lower than the level authorized in some food or feed (400 ppm, USEPA, 1998).”
Professor Séralini’s study published in Environmental Sciences Europe found that both GM maize and Roundup act as endocrine disrupters, and their consumption resulted in female rats dying –at a rate two to three times higher than the control animals. The pituitary gland was the second most disabled organ and the sex hormonal balance was modified in females fed with the GMO and Roundup treatments.
Disruption of Metabolic Pathways
One of the most significant studies was published by Samsel and Seneff in the peer-reviewed scientific journal Entropy in 2013. This comprehensive review, titled “Glyphosate’s Suppression of Cytochrome P450 Enzymes and Amino Acid Biosynthesis by the Gut Microbiome: Pathways to Modern Diseases,” showed how glyphosate disrupted numerous biochemical pathways within the human body, including gut microorganisms, and consequently could lead to numerous diseases.
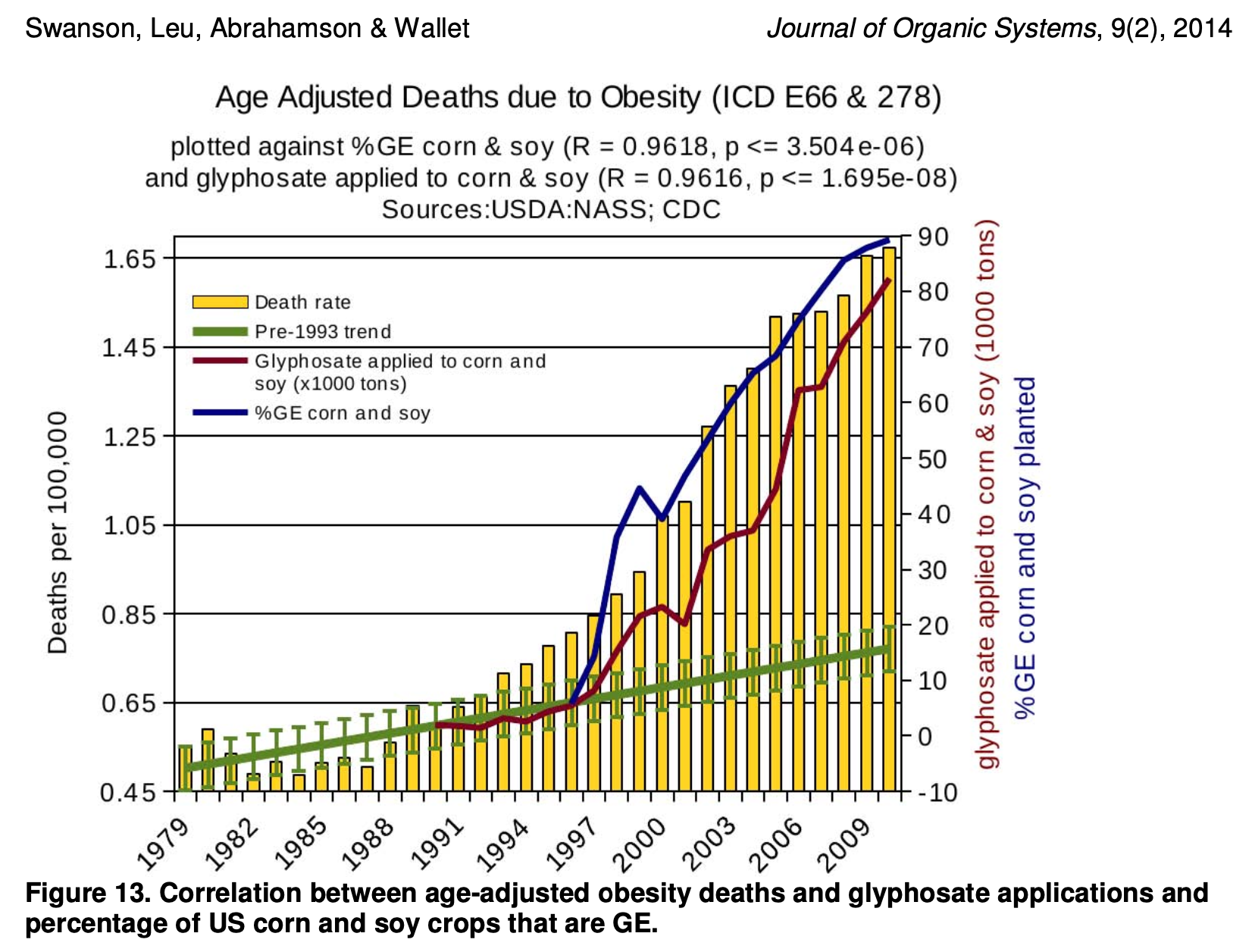
Studies show that disruptions of the normal hormone and metabolic pathways are major causes of obesity, in that they disrupt the normal control mechanisms that stop overeating. Science clearly shows that glyphosate is one of these chemicals.
Diabetes
The rise in diabetes is directly linked to obesity. Most obese people end up with diabetes due to overloading the hormonal mechanisms that regulate blood sugar. Over time they begin to fail, resulting in dangerous increases in blood sugar.
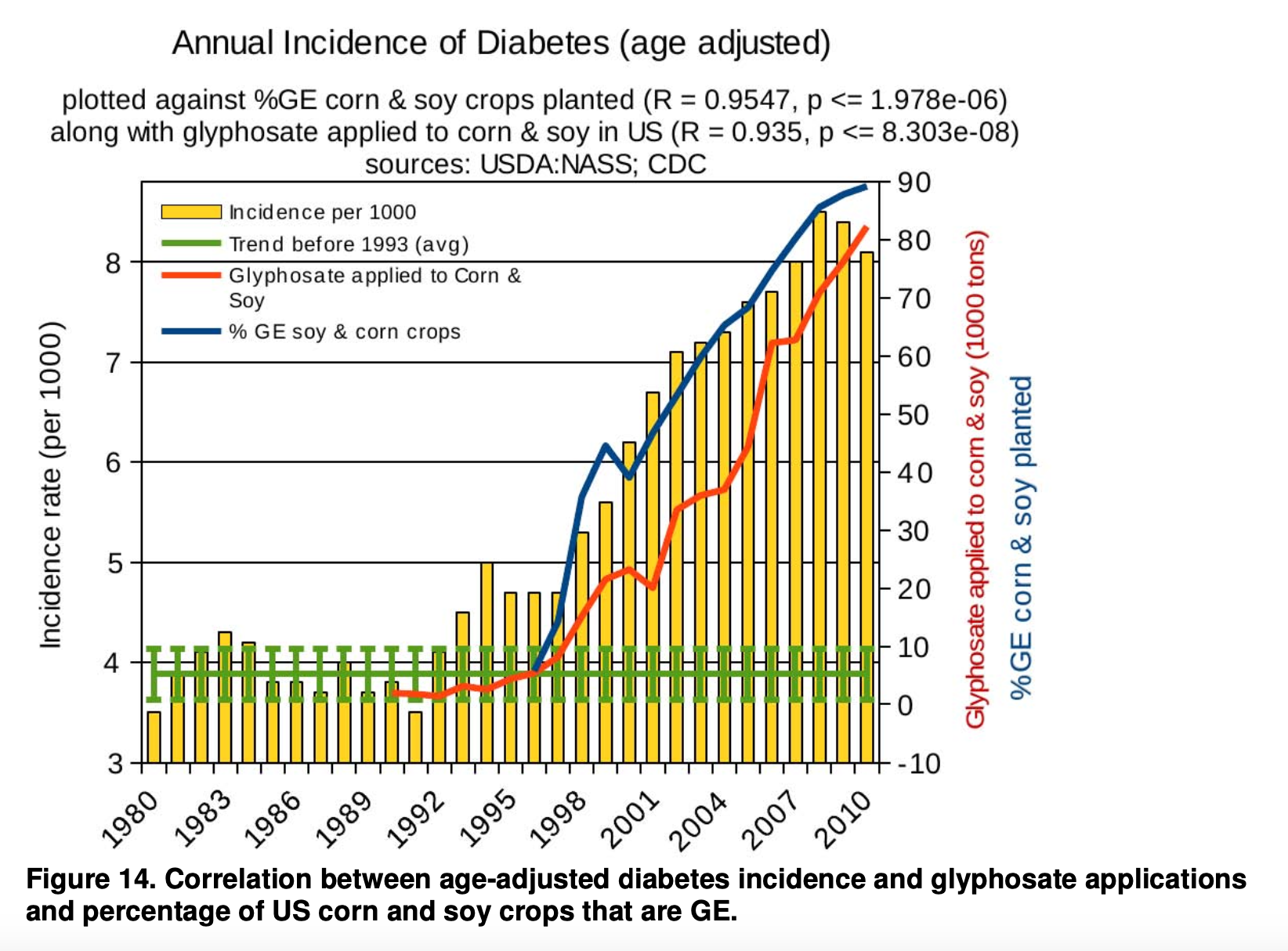
Disruption of the Gut Microbiome
Samsel and Seneff’s paper identified how glyphosate disrupted the gut microbiome, causing the suppression of biosynthesis of cytochrome P450 enzymes and key amino acids. In a later paper, “Glyphosate, Pathways to Modern Diseases II: Celiac Sprue and Gluten Intolerance,” Samsel and Seneff showed that the current increase in celiac disease and gluten intolerance in people was linked to glyphosate’s adverse effects on the gut microbiome. They highlighted that glyphosate is patented as a biocide, and consequently, it kills the beneficial gut bacteria, leading to a rise in intestinal diseases.
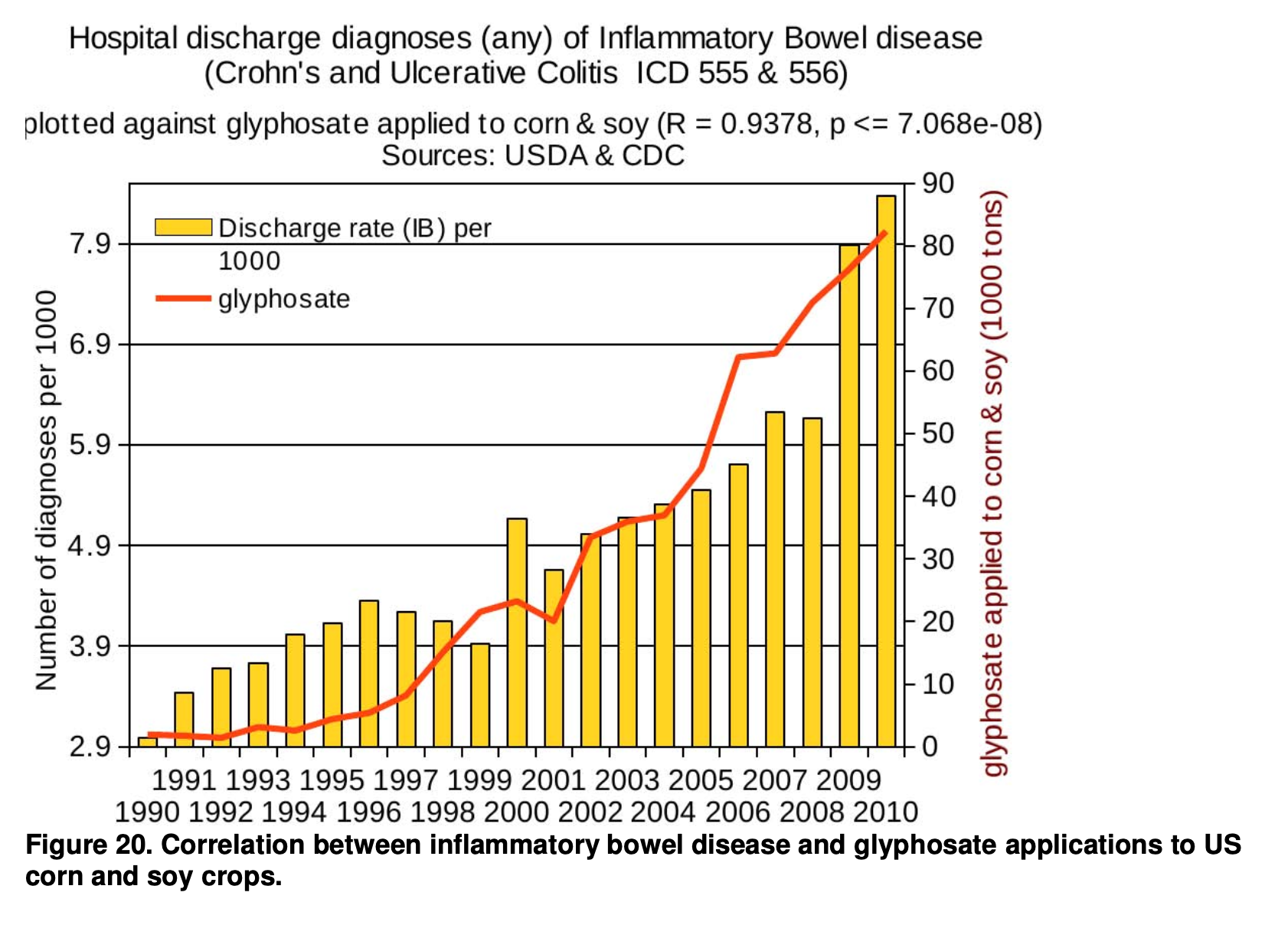
Krüger et al. showed that glyphosate affects the microbiome of horses and cows. Shehata et al. found the same effects in poultry; the researchers state, “Highly pathogenic bacteria such as Salmonella Entritidis, Salmonella Gallinarum, Salmonella Typhimurium, Clostridium perfringens and Clostridium botulinum are highly resistant to glyphosate. However, most of beneficial bacteria as Enterococcus faecalis, Enterococcus faecium, Bacillus badius, Bifidobacterium adolescentis and Lactobacillus spp. were found to be moderate to highly susceptible.” Both groups of researchers postulated that glyphosate is associated with the increase in botulism-mediated diseases in these domestic farm animals.
Inflammatory bowel diseases are rising along with deaths from intestinal infections. Glyphosate’s disruption of the gut microbiome must be seen as a significant cause.
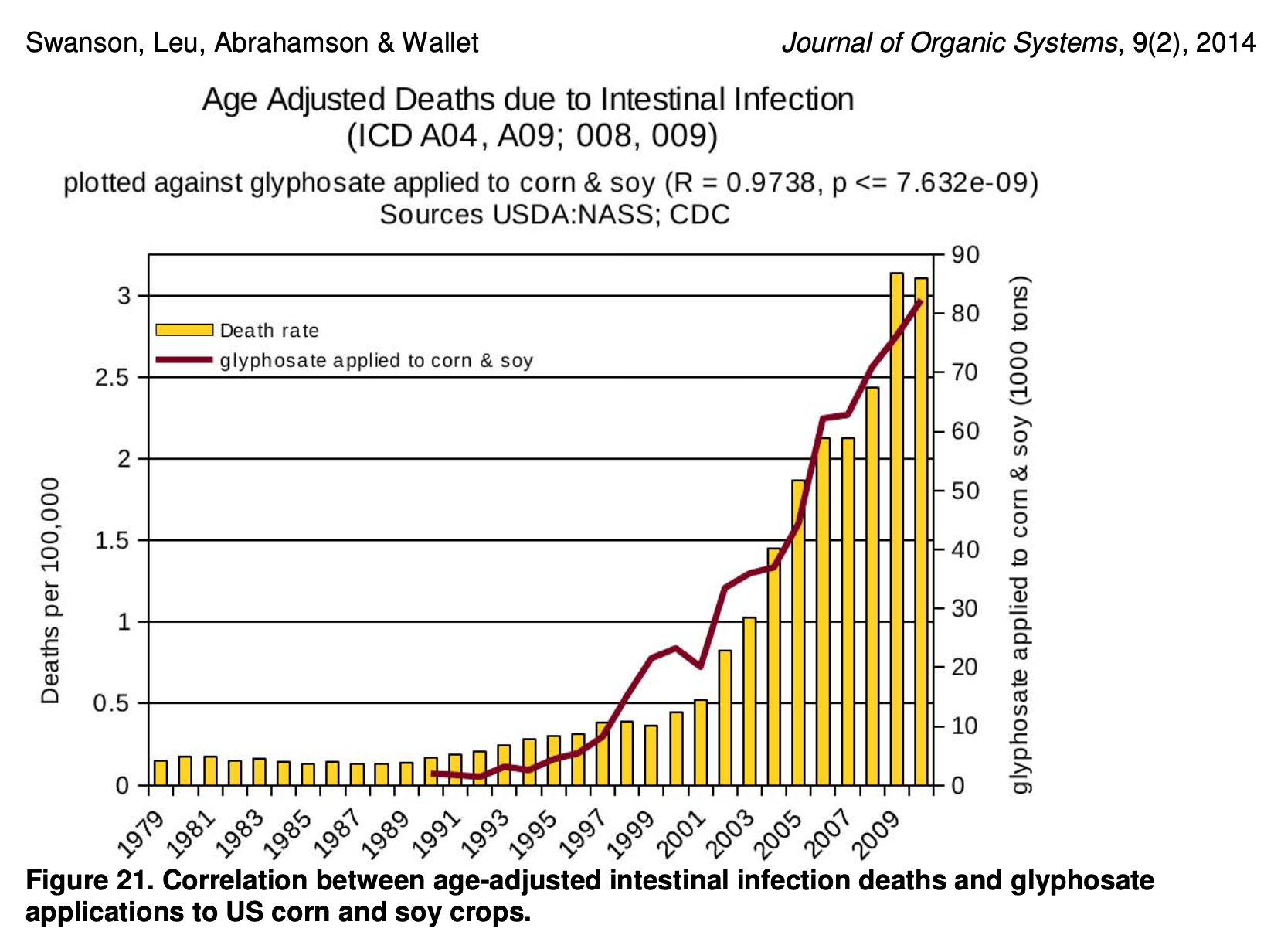
Kidney and Liver Disease
Kidney and liver diseases are major chronic diseases. The graph below clearly shows the relationship between GMOs, glyphosate, and the rapid increase in deaths from kidney disease in the U.S. Deaths from kidney disease fell until the widespread increase of glyphosate and GMOs.
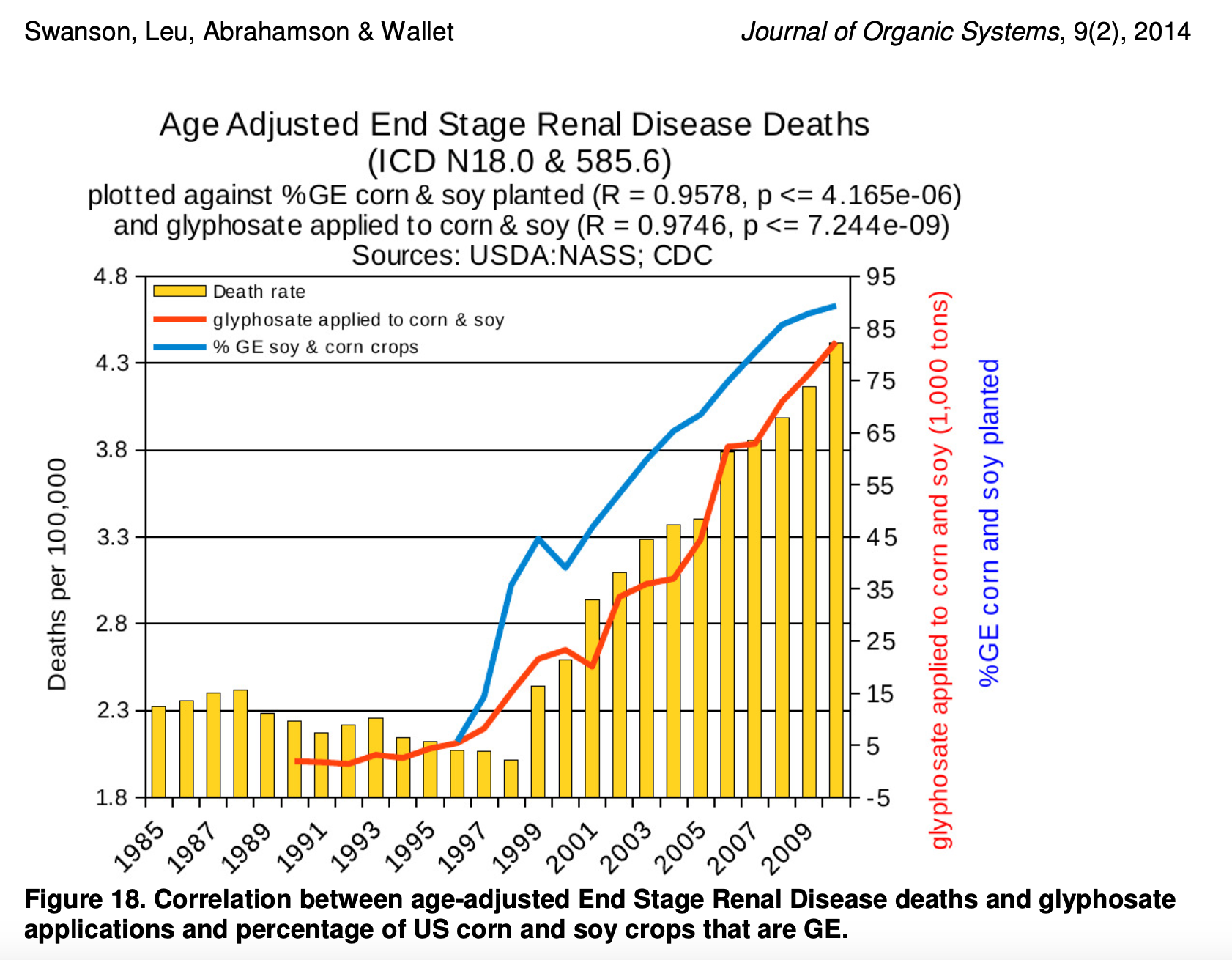
In the lifetime feeding study of rats conducted by Séralini et al. the treated males displayed liver congestions and necrosis at rates 2.5 to 5.5 times higher than the controls, as well as marked and severe kidney nephropathies (kidney damage) at rates generally 1.3 to 2.3 greater than the controls.
The image above shows kidneys and livers that have been damaged by Roundup (glyphosate), GMO corn, and both. In a later published study designed to understand why Roundup and glyphosate-based herbicides caused kidney and liver damage in rats, scientists discovered that ultra-low doses of these herbicides disrupted numerous genes’ functions, resulting in changes consistent with multiple kidney and liver disease problems.
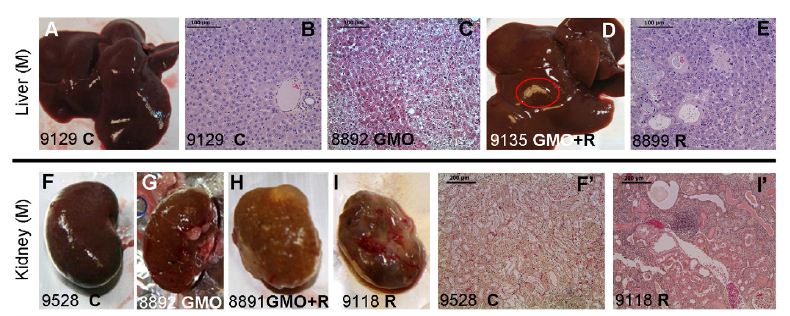
The researchers stated, “Our results suggest that chronic exposure to a GBH (glyphosate-based herbicides) in an established laboratory animal toxicity model system at an ultra-low, environmental dose can result in liver and kidney damage with potential significant health implications for animal and human populations.”
Conclusion
Science shows that GMOs and glyphosate cause multiple chronic severe diseases in the United States. Instead of bullying Mexico to accept these dangerous products, the U.S. regulatory authorities should do their jobs to protect the American people from the harm they cause by banning them.